Plant Genetics and Epigenetics
Explore below for some current projects in the lab. We use genetic, genomic, computational, synthetic, and evolutionary approaches to answer fundamental questions in plant epigenetics and seed biology.
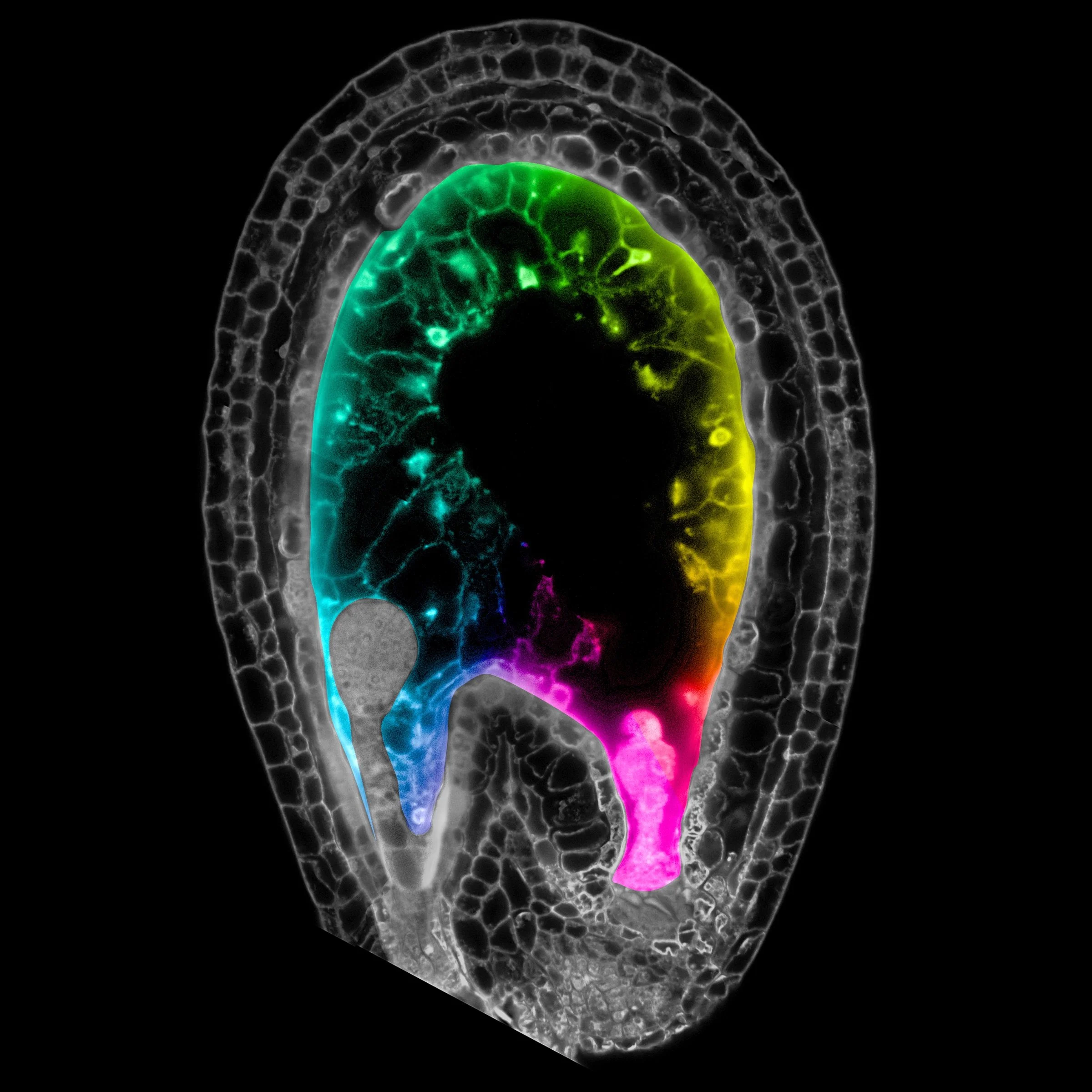
Genetic Conflicts: Evolution, Regulation, and Function of Gene Imprinting
Seeds are the basis of agriculture. They are genetically complex structures with two offspring tissues, embryo and endosperm, surrounded by a maternal seed coat. Endosperm acquires nutrients from the maternal parent and transfers them to the embryo or emerging seedling, like a mammalian placenta. It is triploid in most species, with two maternal and one paternal genomes. Two-thirds of the calories humans consume come directly from endosperm.
We first became interested in seeds because the endosperm is the site of an epigenetic phenomenon called gene imprinting. Imprinted genes are those where alleles are expressed differently depending on whether they are inherited from the male or female parent. Maternally and paternally inherited alleles exist in distinct epigenetic states in the endosperm. DNA demethylation by the 5-methylcytosine DNA glycosylase DME before fertilization is important for establishing imprinted expression and Polycomb Repressive Complex2 is important for maintenance.
Over the years we have explored the function, mechanisms, and evolution of imprinting. The Gehring Lab has identified all imprinted Arabidopsis genes and found those conserved among angiosperms, which likely represent key regulators of seed development. We showed that there is standing variation in imprinting in recently diverged genotypes due to intraspecific DNA methylation variation, suggesting that epialleles may have the biggest impact on plant phenotypes during seed development, when DNA methylation is most dynamic. By comparing imprinting in two closely related species that differ in breeding strategy, we showed that parental genetic conflict appears to underlie the evolution of imprinted expression. We have also shown that imprinted expression is highly conserved between species, but the epigenetic mechanisms governing imprinting can diverge on short evolutionary time scales, suggesting that imprinting in particular genes is likely selected for, rather than being a byproduct of epigenetic reprogramming. Most recently we have investigated imprinting at single-nucleus resolution and shown that it is heterogenous among cell types and varies based on cell cycle phase, suggesting that current models for the establishment and maintenance of imprinting are inadequate. We found that the most parentally biased expression of imprinted genes occurs in the chalazal endosperm (CZE), the region (pink in background image) that interfaces with maternal tissue for nutrient transfer. Using cell/nuclei-type specific profiling, we are currently testing two hypotheses for nuclei-type specific imprinting. Do methylation-sensitive transcription factors (TFs) expressed in the CZE preferentially bind methylated DNA to activate transcription? Or are paternal alleles in the CZE in an epigenetic state distinct from other endosperm regions? We are also keen to understand the function of other, non-imprinted genes that are specifically expressed in the chalazal endosperm, such as small peptides. This work is funded by the NIH and the Manton Foundation.
Function of RNA Pol IV during Seed Development
We are determining how a specialized RNA Polymerase, RNA Pol IV, influences transcription and regulates genome dosage in Arabidopsis. Maintaining the correct dose of gene products and proper gene expression programs is critical for normal development across a range of plant and animal species. We have shown that the RNA Pol IV pathway regulates transcription of maternal and paternal genomes during Arabidopsis thaliana seed development, via both parental effects and in seeds after fertilization. RNA Pol IV is a plant DNA-dependent RNA Polymerase required for the biogenesis of 24 nt small RNAs, the most abundant class of small RNAs in flowering plant genomes. Typically, these small RNAs arise from transposable elements (TEs) and direct DNA methylation to matching genomic regions, leading to transcriptional gene silencing of targeted loci, in a process termed RNA-directed DNA methylation (RdDM). For several years we have been investigating the function of NRPD1, which encodes the largest subunit of RNA Pol IV, in parental conflict, reproduction, and endosperm development. We previously demonstrated that NRPD1 mediates transcriptional balance between maternally and paternally-inherited endosperm genomes by restricting, through an unknown mechanism, maternal genome expression in endosperm. More recently, we also described a function for NRPD1 in crosses between parents that differ in ploidy – paternal inheritance of mutations in nrpd1 suppress seed abortion in interploidy crosses where the paternal dosage is high (e.g. diploid females x tetraploid males), allowing the production of viable triploid seeds. Our most recent paper suggests that NRPD1 has distinct functions in the male and female parents, resulting in antagonistic effects after fertilization. We propose that RNA Pol IV has critical functions during endosperm development that are distinct from the TE-silencing function observed in vegetative tissues. To build comprehensive understanding of the function and molecular mode of action of the RNA Pol IV pathway during reproduction, we are investigating how RNA Pol IV regulates maternal and paternal transcriptional programs in Arabidopsis endosperm, determining the genetic and molecular basis for antagonistic parental effects of RNA Pol IV, and investigating the biophysical properties of Pol IV. This work is funded by the NSF.
Balancing methylation and demethylation activities to maintain epigenetic homeostasis
Eukaryotic genomes must ensure stable inheritance of epigenetic states through cell division both within and between generations. DNA methylation is heritable epigenetic mark essential to maintain transcriptional gene silencing, transposable element silencing, repression of meiosis, and regulation of rRNA expression. In flowering plants, DNA methylation heritability is countered by 5-methylcytosine DNA glycosylases, which remove 5-methylcytosine by base excision repair, leading to its replacement with cytosine. In the Arabidopsis genome, four genes encode 5-methylcytosine DNA glycosylases: DME, ROS1, DML2, and DML3. DME is an essential gene that is maternally required for seed viability, whereas mutations in the others are viable singly or in any combination. We found that stable inheritance of patterns of DNA methylation depend on the balance of methylation and demethylation activities in the genome. ROS1 expression is regulated by both methylation and demethylation in a self-reinforcing negative feedback loop that is necessary for stable transgenerational inheritance of methylation patterns. Importantly, analogous mechanisms have independently evolved in flowering plants and mammals to stabilize the epigenome, and our findings about epigenetic homeostasis in Arabidopsis can be applied conceptually to other systems. More recently, we have side-stepped the reproductive lethality of dme mutants to create Arabidopsis lines that are null for all four demethylases in somatic tissues, allowing us to identify their targets. We showed that active demethylation in somatic tissues plays an important role in maintaining tissue-specific epigenetic states and may regulate flowering time. Currently, we are working to understand cell-type and tissue specific targeting of DNA demethylation. This work is funded by the NIH.
Engineering apomixis
Apomixis refers to asexual (clonal) seed production. We are interested in taking the knowledge we have gained by investigating sexual endosperm development to potentially engineer autonomous endosperm development. We are taking both genetic and synthetic biology approaches. This work is funded by MIT Climate Change Grand Challenges, The Manton Foundation, and the Dr. Vincent J. Ryan Orphan Plant Project.
Underutilized Crops
As the world enters an unprecedented era of climate instability, maintaining an adequate food supply is a pressing challenge. To meet global food security needs, it is estimated that food production must double by 2050, all in the context of increased environmental pressure and competing land uses. Although yield gains have been dramatic over the last 50 years, these have occurred primarily in the staple grains: wheat, rice, and corn. This has been accompanied by a homogenization of the global food supply, with only 50 crops providing 90% of calories consumed globally. Yet, there are at least 3000 plants species which can be grown and consumed by humans. Many of these species thrive in more marginal soils with fewer agricultural inputs, at higher temperatures, and with less rainfall. These underutilized crops are important food sources for farmers around the globe. By definition, underutilized crops have been the subject of little scientific research or breeding programs and there has been little selection for desired traits or increased yield in these plants. Bringing underutilized crops into the molecular age will be vital for addressing current and future food needs. We have begun developing tools and approaches for the underutilized crop pigeon pea (background image). This work is funded by a Bose grant, JWAFS, The Manton Foundation, and the Dr. Vincent J. Ryan Orphan Plant Project.